Biophysics
Daniel Kim-Shapiro, Martin Guthold, Keith Bonin, and Jed Macosko, together with visiting assistant professor Stephen Baker, and George Holzwarth direct research in experimental biophysics. Sam Cho and Fred Salsbury direct programs in computational biophysics.
Daniel Kim-Shapiro leads a laboratory that uses a variety of biophysical and biological techniques to understand blood flow.
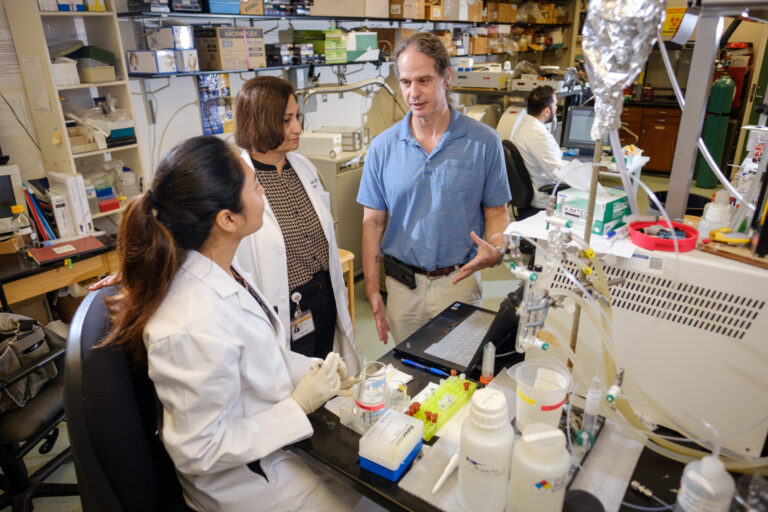
Various spectroscopies are employed including electron paramagnetic resonance spectroscopy, laser diffraction ektacytometry, and time-resolved absorption spectroscopy. Some computational approaches are also employed. The lab studies how the important signaling molecule nitric oxide is compromised in various disease states like sickle cell disease, transfusion of old stored blood, and various cardiovascular disorders. Nitric oxide increases blood flow, decreases platelet activation and circulating blood cell adhesion to the endothelium and can generally reduce inflammation. Therapeutic approaches center on the molecule nitrite which is converted to nitric oxide preferentially under low oxygen conditions. The Kim-Shapiro lab and colleagues hypothesize that nitrite bioactivation involves a novel function of hemoglobin, the oxygen carrying molecule in the blood. Studies include those in silica, in vitro, and in vivo (both in animal and human models) with the aim of establishing a new paradigm in hemoglobin and nitric oxide biology as well as using nitrite in therapeutics.
The research interests of Dr. Guthold’s lab are in the general areas of biophysics, molecular biology, nanotechnology, microscopy techniques, – especially atomic force microscopy (AFM) and fluorescence microscopy, and next generation sequencing. Currently, there are five major projects in his lab: 1) Mechanical and structural properties of fibrin fibers and blood clots. Blood clots perform the mechanical task of stemming the flow of blood. We are using a combined atomic force & fluorescence microscope to determine the mechanical and physical properties of fibrin fibers, which are the principal structural component of a blood clot. We are also using permeability assays, turbidity and light scattering assays and confocal microscopy to gain structural information about fibrin clots. This work will advance our understanding of thrombotic diseases, such as heart attacks, strokes and embolisms, and it may yield novel treatment options for these diseases. 2) Physical properties of cancer cells and normal cells. Recent research indicates that an intricate interplay between genetic mutations and environmental cues, rather than genetic mutations alone, affect cell behavior and fate. We are determining the mechanical properties of normal (non-cancerous) cells, how they compare with cancerous cells, and especially how these properties change as a function of cell micro-environment. 3) Development of a next generation sequencing machine-based drug discovery method. In recent years, only about five new drugs have entered the market per year. Better drug discovery methods may increase this rate. Dr. Guthold’s lab, in collaboration with a team of Wake Forest University Physicists and a company (NanoMedica, LLC) is developing a novel, fast, high-throughput drug discovery technology. The technology utilizes a next-generation sequencing chip and nucleic acid-encoded chemicals. 4) AFM imaging of biological samples, nanofibers and thin films. We are using AFM to image and obtain structural information of protein-DNA complexes, drug-treated DNA molecules, nano-fibers and thin films. Collaborators are welcome to use his instruments. 5) Properties and utilization of electrospun nanofibers. Cells are very sensitive to the mechanical properties and the geometry of their substrates. We are determining the mechanical and structural properties and structures of several nanofibers that are used for tissue engineering and other applications.
Keith Bonin’s research has been focused on optics and biophysics, and consists of collaborations with other professors within the Physics Department or at the Wake Forest School of Medicine (WFSOM). Currently research includes:
- mechanical properties of cells in 2D;
- optogenetics studies of the mesolimbic system in the brain;
- studies on the motion of double-strand breaks in DNA in cancer cell nuclei; and
- studying structural properties of electrospun fibers that can be used as tissue scaffolds.
Project 1 on mechanical properties of cells, in collaboration with Professor Guthold, involves several optical and physical techniques to understand cell mechanics, and the role of cell-cell signaling on multicellular systems, specifically those that involve cancer cells. Project 2 on optogenetics in the brain, in collaboration with Profs. Evgeny Budygin and Jeff Weiner at WFSOM, involves using the tools of optogenetics to study the connections of the important components of the reward system in human brains, namely the nucleus accumbens and the ventral tegmental area. Studies are currently focused on behavioral studies in rats to understand and reduce alcohol consumption using optogenetics. Project 3 on the motion of double-strand breaks (DSBs) in DNA, in collaboration with Prof. Pierre Vidi-Alexander at WFSOM and Prof. G. Holzwarth, is focused at trying to model the behavior of the ends of the DNA at the cut site, and to understand whether the motion in different regions leads to different amounts of translocation that can result in mutations. This is important in understanding the effects of cancer radiation treatment in forming secondary tumors. Project 4 focuses on generating cellular/tissue scaffolds made by electrospinning fibers made of combinations of natural proteins and a synthetic, biodegradable polyester – polycaprolactone (PCL).
Jed Macosko’s group focuses on understanding the mechanics of protein machines. They use atomic force microscopy (AFM), single molecule fluorescence microscopy and video-enhanced differential interference contrast light microscopy (VE-DIC), to study how protein motors use chemical fuel to power their work cycle. They use viscous drag as well as magnetic forces to slow down or speed up the movements of the motors. This gives information about the potential energy surface corresponding to the rate limiting mechanical transition in the molecular motor. Their long-term goal is the identification of precise mechanical-chemical couplings in molecular machines and the characterization of the overall pathways of their physical motion. Together with George Holzwarth, Macosko’s group is also studying the mechanical properties of live cells in 2D culture. We track the motions of fluorescently tagged peroxisomes in live normal, tumorigenic and metastatic human breast cells. From the tracks, we can extract the elastic and viscous shear moduli of the cytoplasm. These moduli play a role in the dynamics of cell deformation, which occurs during metastasis.
Durotaxis and mechanical properties of live cells grown in 3D culture. We are growing cells within collagen gels which are cross-linked by photogenerated free radicals. We plan to using an optical mask to generate, gels with a spatially varying elastic modulus in a physiologically useful range to study durotaxis.

George Holzwarth collaborates with Keith Bonin and Jed Macosko, as described above. Independently, he has used variational Bayes probability methods to analyze the cause of start-stop movement of endogenous particles, such as peroxisomes, in live cells. Holzwarth is currently collaborating with Pierre-Alexandre Vidi (Cancer Biology, Wake Forest University School of Medicine) and Keith Bonin to study the mobility of GFP-labeled histone 2a in the nuclei of live cells during DNA repair. Chromatin mobility is determined by subpixel tracking of photo-activated, diffraction-limited GFP spots.
Sam Cho’s interdisciplinary research group interests encompass biophysics and computer science. As such, their group consists of undergraduate and graduate students with physics, computer science, chemistry, biology, and mathematics backgrounds. They are interested in the theoretical and computational studies and methods development of biomoleular coarse-grained and atomistic molecular dynamics (MD) simulations in collaboration with experimental groups. The inherent computational complexity of MD simulations, however, require cutting-edge high performance computing to perform simulations of biologically relevant sized systems for timescales that correspond to biological functions. One of our primary focuses is to develop MD simulation approaches using graphics processing units (GPUs), which are ideally suited for parallel algorithms such as those in MD simulations.
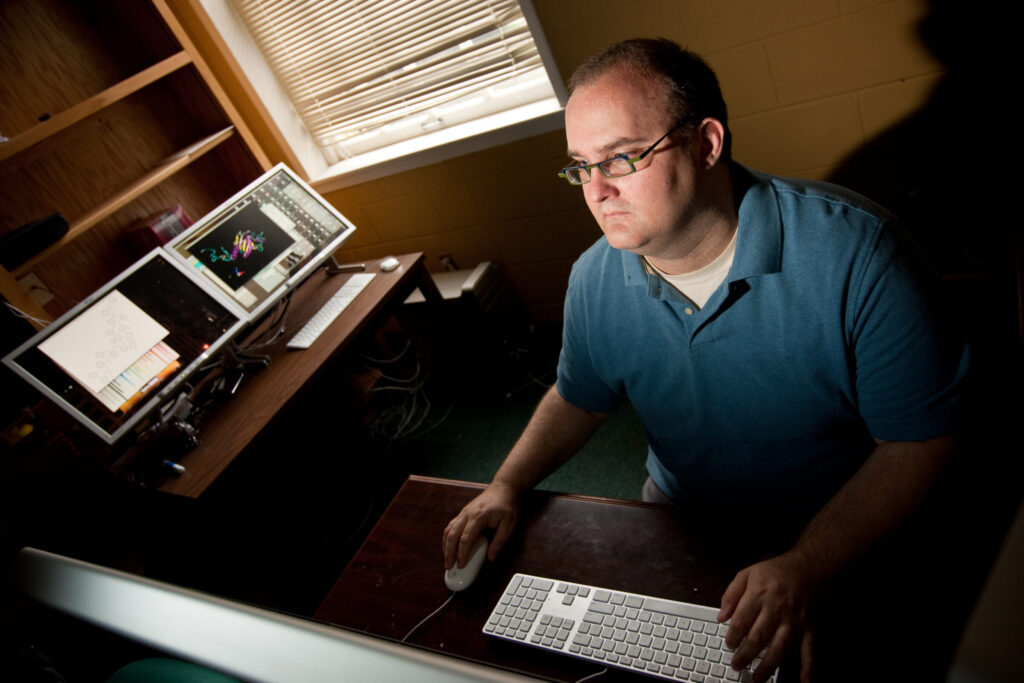
Fred Salsbury‘s research aims to help in the fight against disease by applying tools from computational physics to molecular-scale problems. Biomedical advances increasingly require interdisciplinary approaches and computational tools are increasingly able to address the time-scales and length-scales necessary to be a part of these advances. We focus on the molecular scale, using tools from statistical and computational physics, to study problems from the basic to the applied in the development of cancer therapeutics. We work at the interfaces between the life and physical sciences, and within the physical sciences, between chemistry and physics. Specific current projects focus on: 1) microsecond-scale all-atom molecular dynamics simulations with GPUs 2) virtual screening for drug discovery in collaboration with colleagues in cancer biology, chemistry and biochemistry, and 3) development and application of new statistical methods to analyze and understand molecular dynamics data. 4) application of machine learning to analysis and prediction of macromolecular dynamics and drug development.